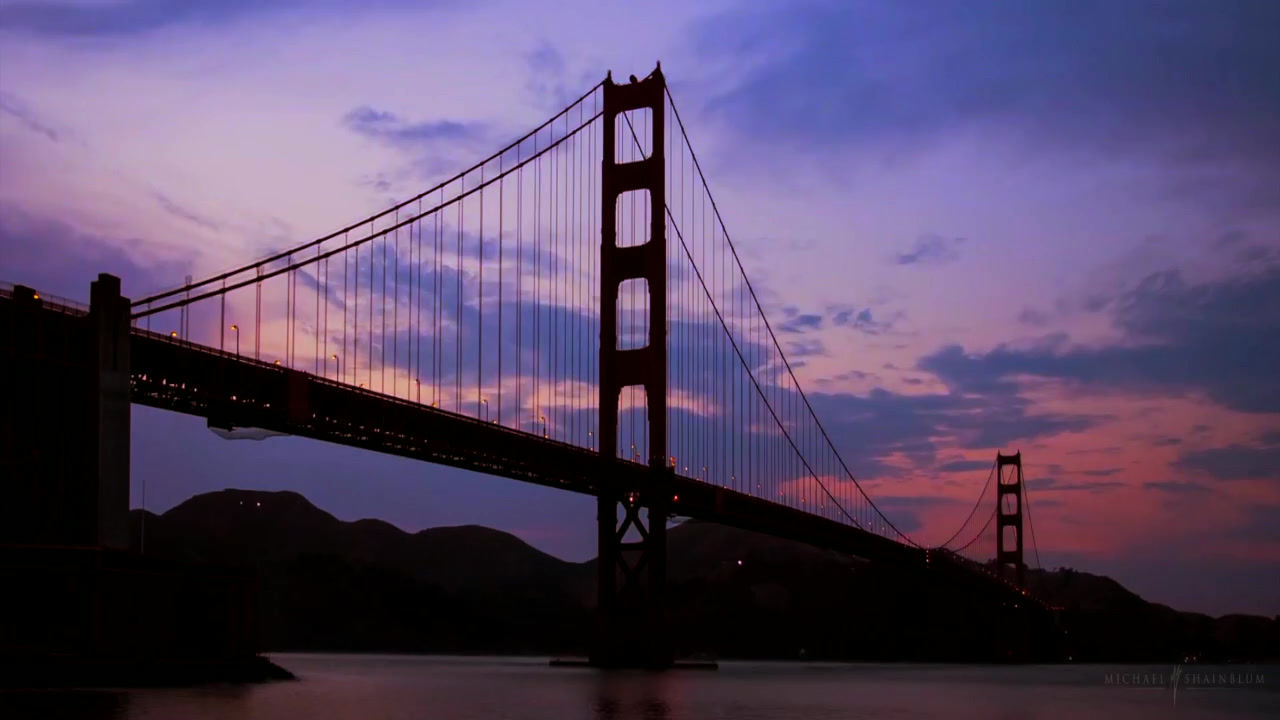
As the 20th century drew to a close, biotechnology’s equivalent of Promethean fire appeared: the first detailed sequence of the human genome. Now, the ability to decrypt genetic sequences has empowered us - albeit while creating significant risks. DNA can now be picked apart and rearranged like a bouquet of flowers.
The DNA structure discovered by Watson and Crick in 1953 begat a restriction enzyme that can cleave DNA within seconds, which begat the discovery of biochemical enzymes that process DNA, which begat gene-editing technology that could be used to directly control the expression of intracellular genes within a nucleus. However, it was neither cost-effective nor efficient to manipulate gene expression within the nucleus - a problem solved by the breakthrough discovery of the CRISPR-Cas9 system in the early 21st century.
Researchers found that by attaching a specific sequence of guide RNA joined up with a protein called Cas9 to a genome, they could cut and delete (or add) DNA. Soon after its discovery, CRISPR-Cas9 became a global sensation due to its relative ease and compatibility with just about any type of organism.
Genome manipulation techniques were eventually discovered that can eradicate or correct erroneous genes, to create recombinant embryos, treat cancer, or prevent infectious diseases. In the near future, gene therapy drugs could be injected, potentially eliminating the need for surgery to avoid genetic defects - such as the procedure the actress Angelina Jolie underwent to address her predisposition to breast and ovarian cancer.
A Chinese scientist’s successful effort in 2018 to create genetically-engineered babies in order to endow them with a resistance to their father’s HIV infection shocked the scientific community and points to a need for greater global awareness of the perils of genetic engineering technology as a means of artificial reproduction (Chinese officials have since declared that the scientist’s conduct was illegal). Prometheus's gift, after all, had serious repercussions for civilization.
Epigenetics, or the study of genetic expression that doesn’t involve changing a DNA sequence, promises future innovation for genome engineering. Epigenetics could eventually enable humans to adapt to, and evolve in, environments such as the atmosphere of Mars. However, genome engineering comes with ethical issues.
Bio Big Data and Machine Learning



Biomedical science is transforming into big-data science. The automated analysis of increasingly large sets of genetic data promises to transform health care. Thanks to next-generation genomic sequencing technology, there has been a dramatic data explosion; as of 2016, more than 100,000 human genomes had been sequenced from normal and diseased tissue, and petabytes of raw sequence data are now being produced and deposited in public genome data repositories such as International Cancer Genome Consortium data portal.
This is transforming the scientific landscape, and entire healthcare systems. Currently-archived datasets represent only a small fraction of the genome-related big data yet to be produced, as sequencing capacity will continue to grow. If the current growth rate continues, doubling capacity about every seven months, exabytes-worth of genome data (one exabyte is equivalent to about 250 million DVDs worth of video) will be yielded in the next 5 years.
As the global population pushes toward 8 billion within the next decade, it is possible that 15% or more of it will have their genome sequenced. In addition to genomics, high-resolution imaging, medical records, and lifestyle-related datasets will add new dimensions to bio big-data, and provide a foundation for next-generation healthcare.
However, there is a long road ahead before big data can help deliver precision medicine - based on a patient’s genetic makeup and environmental circumstances - to the masses. There are immense challenges when it comes to data storage, distribution, and proper interpretation in biomedical contexts.
Machine learning could facilitate the mining of gene-to-gene interaction, the classification of cellular images, and finding links between datasets. Yet, machine-learning algorithms require large-scale, high-quality “ground truth” data for algorithm training - which is difficult to acquire. Large-scale machine learning systems need to be integrated with vast computing infrastructure in order for deep learning, one of the most promising branches of artificial intelligence, to help better enable the navigation of big data and detect things that are impossible to catch manually.
In addition, machine learning can be biased, and understanding exactly how machine learning algorithms are classifying the features in datasets can be challenging. Still, the analysis of big data will eventually have an enormous impact on disease prevention, on the ability to cure and care, and on the global healthcare system.
Medical Biotechnology
Ageing populations and people with genetically-inherited diseases can benefit from gene editing, but there are ethical concerns. Medical biotechnology is a rapidly developing means to rejuvenate the elderly and regenerate ageing or diseased organs - a potentially helpful development for the many countries with greying populations (nearly 8.7% of the global population was aged 65 or older by 2017, up from 6.7% in 1997, according to World Bank data).
The use of “induced pluripotent” stem cells, which do not have to be derived from human embryos, has shown promise for regenerating an injured heart (while avoiding the ethical issues tied to the use of human embryonic stem cells), for example. Biotechnology tools are also being used for the diagnosis and treatment of human disease in a personalized way.
We are currently able to modulate each layer of biology, from genes to proteins and cells. When it comes to the immunological treatment of cancer, the clinical development of protein- or antibody-based therapeutics is advancing, and the range of biological targets is expanding. In addition, genetically modified immune cells are being developed as immuno-oncology drugs, including chimeric antigen receptor (CAR)-T cells and T-cell receptor (TCR)-transduced T cells.
Regenerative medicine will be accelerated by gene-editing technology. CRISPR-Cas9, for example, enables the direct modification of genes in the interest of preventing and treating disease - though there are significant ethical issues related to altering the human genome in ways that will be passed from one generation to the next (a Chinese scientist stunned the world in 2018 when it was revealed that he had engineered the world’s first gene-edited babies in a bid to make them resistant to their father’s HIV infection).
Personalized cancer vaccines, and drug tests using surrogates, are also major elements of precision medicine. Tissue engineering has also evolved, thanks to organoid (a miniaturized organ produced in vitro) technology, novel biomaterials, and 3D printing. In general, a more personalized application of medical biotechnology is necessary, to enhance therapeutic efficacy and minimize unwanted side effects. And, the most important personal factor is the genome - in terms of genetic and acquired diseases.
Integrating genome information into medical data is an important component of precision medicine, and is being aided by artificial intelligence.
Synthetic Biology

Microbial cell factories can sustainably produce the chemicals needed to fuel an economy. Civilization has largely committed itself to forging a more resource-efficient and sustainable global economy.
One of the ultimate goals is to reconcile demand for chemical materials needed to treat human disease, develop sustainable agriculture and fisheries, bolster food security, and power industrial applications with the need to ensure biodiversity and environmental protection.
In order to help achieve this, many countries have leveraged advances in synthetic biology and metabolic engineering by using natural biological processes to produce important chemicals - and by making use of standardized, intelligent “cell factories” (collections of microbial cells, often built from bacteria or yeast, that can function like a chemical production facility).
The consequences of this could be as significant as the impact of alchemy on chemistry millennia ago, with enormous and as perhaps unimaginable implications for medicine and materials science. The range of potential applications is vast, encompassing, and not limited to: diagnostics, therapeutics, sensors, environmental remediation, energy production, and biomolecular and chemical manufacturing. Studies have shown, for example, the potential to deploy bacteria-based cell factories to sustainably produce ethanol and butanol - which could in turn be used to de-carbonize transportation.
Following the emergence of recombinant DNA technology, which mashes up DNA from different species to produce combinations that have value for medicine or industry, biological systems have become widely used in industries such as chemicals and pharmaceuticals. However, the cell factories underpinning these processes often encounter systemic failure and suffer from instability.
In 2016, systems metabolic engineering was selected as one of the Top 10 emerging technologies by the WEF, for the ways that it can be used to more sustainably and affordably produce chemicals by using plants instead of fossil fuels. Synthetic biology and metabolic engineering can be applied to these problems by developing cells specifically designed for predictable, efficient, and streamlined production.
Synthetic biology and metabolic engineering also enable the development of new biological systems capable of efficiently producing industrial chemicals and materials while consuming relatively less time, labor, and money. Some examples of this trend include reported advances in the microbial production of gasoline, terephthalic acid (an organic compound used to make clothing and plastic bottles), 1,4-butanediol (used to make fibers such as Spandex), and aromatic polyesters.
Industrial Biotechnology

Industrial Biotechnology, also known as “white” biotechnology, involves the sustainable production of chemicals, pharmaceuticals, food, fibres, textiles, and energy. “White” biotechnology can help feed the undernourished and better protect the environment.
White biotechnology is often referred to as the third wave of biotechnology, following “red” biotechnology (medical-related) and “green” biotechnology (agriculture-related), and it has become a powerful means to address climate change, mounting energy demand, the depletion of fossil-fuel resources, and environmental issues including petroleum-based plastics and micro-plastics pollution.
The field’s underpinning technologies are mainly based on the engineering of microbial hosts, and it has been boosted over the course of the past decade by scientific breakthroughs in metabolic engineering, synthetic and systems biology, and advanced cultivation techniques. In particular, the recent establishment of systems metabolic engineering (combining metabolic engineering with systems biology, synthetic biology, and evolutionary engineering) has led to a dramatic advances. Through the comprehensive optimization of existing methods, and the creation of new methods, the spectrum of possible commercial products has been expanded dramatically - including healthy food products that can be used to combat malnutrition in impoverished parts of the world.
According to a study published by the Organisation for Economic Co-operation and Development, The Bioeconomy to 2030: Designing a Policy Agenda, industrial biotechnology will account for 39% of all economic value generated by biotechnology by the year 2030. Compared with traditional, fossil fuel-based chemical processes, an industrial biotechnology process completed via a single fermentation can be a way to make relatively affordable products that help protect the environment.
As a result, the total industrial biotechnology market is expected to grow at a rate of nearly 9% annually, and reach a total size of $472.3 billion by the end of 2025. Bioethylene, for example, is expected to account for as much as 40% of all plastic polyethylene production by 2035 - and production of biodegradable polylactic acid, usable for everything from decomposable packaging material to medical implants, is expected to reach 800,000 tonnes by 2020, according to the International Energy Agency and International Renewable Energy Agency.
Ultimately, industrial biotechnology can make a major contribution to achieving United Nations Sustainable Development Goals related to fostering affordable and clean energy, sustainable cities and communities, and responsible consumption and production.
Environmental Biotechnology
Agricultural Biotechnology

Metabolic and bioprocess engineering can be used to treat waste water and tackle serious environmental problems - including plastic pollution. Plastic pervades our lives while spoiling the environment, and biotechnology may provide a sustainable solution.
The production and incineration of plastic will add more than 850 million metric tons of greenhouse gases to the atmosphere during 2019, equivalent to the pollution caused by 189 coal-fired power plants, according to a report published by the Center for International and Environmental Law.
In 2016, a Japanese research team from the Kyoto Institute of Technology and Keio University published a report in the journal Science about a bacterium called Ideonella sakaiensis, which contains an enzyme, PETase, that was found to be able to degrade PET. Plastics like polyethylene terephthalate (PET) pervade our daily lives because they are cheap to manufacture and highly durable. This durability causes serious damage; as much as 8.8 million metric tons of plastic waste is estimated to enter the ocean every year, where it will remain indefinitely.
Microorganisms, which have mostly been used for the production of chemicals and materials, can also be applied to the biodegradation of plastics in a way that enables a highly-sustainable recycling system.
A Korean research team from the Korea Advanced Institute of Science & Technology (KAIST) and Kyungpook National University further delved into this PET-degrading enzyme and determined its 3D crystal structure, while predicting a molecular mechanism of the PET degradation. They published their research in the journal Nature Communications in 2018 - and the approaches behind it can now be applied to the study of enzymes that could degrade other types of plastics.
One lingering challenge has been to find a way to improve microbial production performance at an economically viable scale - which will need to be overcome in order to significantly mitigate plastic pollution and establish sustainable plastic recycling.
Recent research on the biodegradation of plastics has created potential for synergy with the sort of microbial production of biodegradable polymers that have long been studied by microbial engineers. Microorganisms can now be engineered to produce polyhydroxyalkanoates (polyesters that can store carbon), polylactic acid (a biodegradable polyester), and, as of recently, aromatic polyesters that use renewable biomass feedstock as raw material.
“Green” biotechnology can help generate more nutritious food for an expanding global population. Agricultural, or “green” biotechnology can be used to help improve crop yields and quality, nutrient usage, disease resistance, environmental stress resistance, and flowering time.
The pinnacle of breeding technology has been the development of “semi-dwarf” wheat, which is sturdier and higher-yielding, and now accounts for most of the wheat acreage in the world after appearing around the middle of the 20th century. Advances in DNA technology, coupled with the identification of genes essential for desired traits, raised green biotechnology to a new level; scientists became able to not only pinpoint a gene regulating a specific trait, but also to introduce the gene into plant genomes by using methods such as particle bombardment.
This ability to introduce a specific gene as a DNA fragment removed the biggest hurdle for conventional crop breeding: reproductive isolation separating different species. The successful generation of the blue rose using a blue gene from another plant species, for example, is testament to this.
The removal of this species barrier has opened up new ways to mass produce valuable products, like edible vaccines and medicinal compounds. Meanwhile genome editing technology enabled by ZFN, TALEN, and more recently CRISPR/Cas9, promises even more precise plant engineering. This technology can knock out a specific gene, change the sequence of a specific gene, and replace one DNA fragment with another. This can occur without leaving a trace of foreign DNA or resulting in antibiotic-resistant genes (a flaw often associated with genetically-modified organisms, or GMO).
Technology has been used to modify the genetic content of plants throughout the history of human civilization - long before the discovery of DNA, Neolithic-era farmers developed domesticated crops by selecting those with desirable traits derived from naturally-occurring mutation. Later, the selection of the most desirable plants from the general population was crystalized into the concept of breeding - which spawned a plethora of modern crops.
The recent development of a soybean variety that can produce high levels of heart-healthy oils, and the engineering of wild ground cherries in a way that can make them as domesticated as strawberries, underline the ways green biotechnology may be able to help sustain a rapidly-expanding global population.
BIOTECHNOLOGY

The Biotechnology Services Market Is Expected To Reach $267 Billion By 2026 Due To A Rapid Increase In Lifestyle And Chronic Diseases As Per The Business Research Company's Biotechnology Services Global Market Report 2022.
This is mainly due to unhealthy lifestyles, a growing aging population, rising inactivity and obesity. According to the World Health Organization, chronic disease prevalence is expected to rise by 57% globally by the year 2020. China and India together had about 193.4 million diabetic patients in 2019, and India is expected to have about 101 million diabetic patients by 2030. In the field of mental health, the World Health Organization predicts that one in every four people will be affected by a type of mental disorder such as depression, schizophrenia, or anxiety in the future. In response to this, national governments across the globe are rapidly increasing their medical research expenditure. The world's population is also growing older with every passing year.
Medical biotechnology is personalizing medicine by keying on our individual biology, industrial biotechnology is being used to develop more eco-friendly ways of building things, agricultural biotechnology can help feed an expanding global population, and synthetic biology is helping to sustainably produce essential chemicals and materials.
Meanwhile environmental biotechnology may yet solve the vexing problem of plastic pollution. However, while recent biotechnology-related advances have opened up incredible new possibilities, they have also created significant ethical issues - as society grapples with concepts like gene-edited babies.